All published articles of this journal are available on ScienceDirect.
Validation of a Vibrotactile Stimulation System Using the Wii Remote for Studies of Tactile Sensitivity
Abstract
Background:
Vibrotactile stimuli are widely used to study the functional characteristics of the haptic sense. Although many vibrotactile stimulators are commercially available, most are expensive.
Objective:
We developed a low-cost vibrotactile stimulation system using a Nintendo Wii Remote and a personal computer. In the present study, we examined the validity and accuracy of this vibrotactile stimulation system.
Method:
In the first experiment, we measured the linearity of vibration amplitude and changes in peak vibration frequency generated by the Wii Remote as a function of activation intensity. In the second experiment, the effect of vibrotactile stimuli applied to the dorsal surface of hand on two-point discrimination threshold at the index finger was examined in twelve participants.
Results:
The peak vibration frequency was about 150 Hz irrespective of vibration intensity, which would effectively activate fast adapting type II (FAII) cutaneous mechanical receptors. The two-point discrimination threshold measured at the third pad of the index finger decreased significantly when a near-threshold vibrotactile stimulus was applied to the dorsal hand, a response termed stochastic resonance (SR). These SR results are consistent with findings in the other sensory systems, such as auditory, visual, and somatosensory systems.
Conclusion:
This newly developed stimulation system produces controllable vibrotactile stimuli useful for study of the haptic sense.
1. INTRODUCTION
Vibrotactile stimulation is widely applied in the study of haptic sensory function [1-3] as well as for tactile sensors and displays [4, 5]. Several systems have been developed to generate vibrotactile stimuli, including a motor for repeated touch stimulation [6, 7], a moving magnet linear actuator [8], a voice coil actuator [9], and a piezoelectric actuator [10-12]. In addition, several mechanical stimulators are commercially available. However, most are expensive or a frequency characteristic changes with amplitude. To mitigate this problem, we have developed a low-cost vibrotactile stimulating system using the Nintendo Wii Remote and a personal computer (PC). This wireless device is the man–machine interface for Nintendo Wii and Wii U consoles and is equipped with a small oscillator as well as an infrared sensor and multiple acceleration sensors. The Wii Remote uses Bluetooth for console communication. In our system, we control the Wii Remote oscillator by PC software via Bluetooth. Here we examined the accuracy and experimental utility of vibrotactile stimulation generated by the Wii Remote.
2. EXPERIMENT-1
In the first experiment, we analyzed the linearity and reproducibility of amplitude and the changes in peak vibration frequency generated by the Wii Remote as a function of activation intensity.
3. METHODS
3.1. Apparatus
In order to stabilize the source currents for the Wii Remote (RVL-003, Nintendo), we used DC currents from a USB port (USB feed adopter, CY-WIURUSAD-WH, Cyber) rather than the internal batteries (1.5V × 2). We used a PC running Windows XP and an open-source program (Wiibrator02.exe; [http://www.kako.com/ neta/2006-019/ wii_brator2a.zip] 2016/12/26) to control the Wii Remote via a Bluetooth USB adapter (BT-Mixro3E2X, PCI). The software generates four intensities of vibration as selected by the [+] and [−] buttons, and controls stimulus “on”/”off” by the [A] button. The selected intensity is indicated by LEDs on the Wii Remote. However, in preliminary studies, we found that the time course of the vibration differed among intensity levels. In particular, an irregular beat oscillation was observed at some intensity. Thus, we chose the 4th intensity (maximum) for subsequent studies because the beat is the smallest, and modified output intensity by cushioning sheets as described below.
3.2. Recording of Vibration
The frequency distribution and amplitude of the vibration were measured using an accelerometer (GH313A, GA-245SO, Keyence). The Wii Remote was placed on a small table (45cm wide, 65cm long, and 2cm thick) and the sensor of the accelerometer was affixed on the tabletop 5 cm from the Wii Remote using double-faced adhesive tape to capture traveling vibrations. This accelerometer position was selected as the midpoint between the stimulator (Wii Remote) and the participant’s dorsal hand in experiment 2 (10cm away as described below). The output of the accelerometer was input to an oscilloscope and an A/D converter (MacLab, A/D Instruments) with a sampling rate of 1 kHz. The digitized waveforms were then stored on the hard disk of a PC (Macintosh, Apple). Power spectrograms of the recorded waves were produced by Fast Fourier Transform (FFT) with a sampling number of 1024 (Chart v.3.5.6, A/D Instruments).
3.3. Procedures
As described above, the vibration intensity setting of the software (activation intensity) was fixed at the level producing the smallest beat. We therefore changed the intensity of vibrational output using a variable number of cushioning sheets between the vibrator (Wii Remote) and the table. Specifically, intensity was reduced by increasing the number of sheets from 1 to 4. Thus, this vibrotactile system was designed to apply the stimulus simultaneously to a relatively wide area of the hand when placed on the tabletop 10 cm from the Wii device.
4. RESULTS
Changes in vibration amplitude as a function of output intensity are shown in Fig. (1). The vibration output intensity was changed from level 1 (S1, lowest) to level 5 (S5, highest) by decreasing the number of cushioning sheets between the Wii Remote and tabletop from four to none, respectively. The amplitude of vibration was measured by the accelerometer output voltage. As expected, vibration amplitude increased progressively with output intensity level (Fig. 1), and the relationship was nearly linear.
Fig. (2) shows the frequency power spectra from 0 to 500Hz for all six vibration intensities (S0–S5). The spectral pattern was similar at each non-zero intensity (S1–S5), with peak frequency at about 150Hz. This peak disappeared at S0 (no vibration).
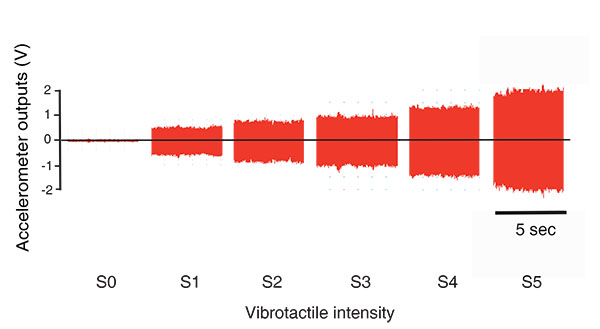
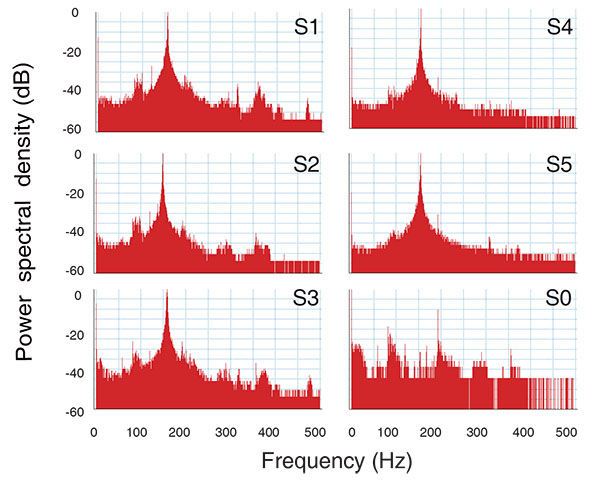
4.1. Experiment-2
In the second experiment, the validity and utility of the Wii Remote were tested in a psychophysical study examining modification of two-point discrimination threshold by vibrotactile stimulation.
Noise is generally thought to hinder signal detection. However, normally undetectable signals can be perceived by addition of weak noise to the signals in a non-linear system, a phenomenon called stochastic resonance (SR) [13]. Improved signal detection by weak noise input has been studied not only in the field of physical events but also in biological systems, including human sensory systems [14-16]. In the human somatosensory system, touch sensation of the hand can be enhanced by weak vibrotactile noise [6, 12, 17]. Collins and colleagues found that detection of a touch stimulus to the hand improved when vibration noise was added to the touch stimulus. In addition, they demonstrated that impaired sensitivity to tactile stimuli to the foot can be reversed by localized low-level electrical noise in patients suffering from peripheral nerve diseases [6, 7]. Kurita et al. [12] found that two-point discrimination threshold at the tip of the index finger was improved significantly when a weak noise stimulus was applied simultaneously to the side of the index finger. Collectively, these studies indicate that tactile sense can be improved by near-threshold noise if applied close to the site of the test stimulus.
In SR, addition of a weak noise around its detection threshold improves subliminal signal detection, while more intense noise either has no effect or impairs detection. Thus, the detection threshold will show a U-shaped function with noise intensity [18-20]. In the present experiment, we examined the effect of remote vibration applied to the dorsal surface of hand on the two-point threshold measured at the third pad of the index finger.
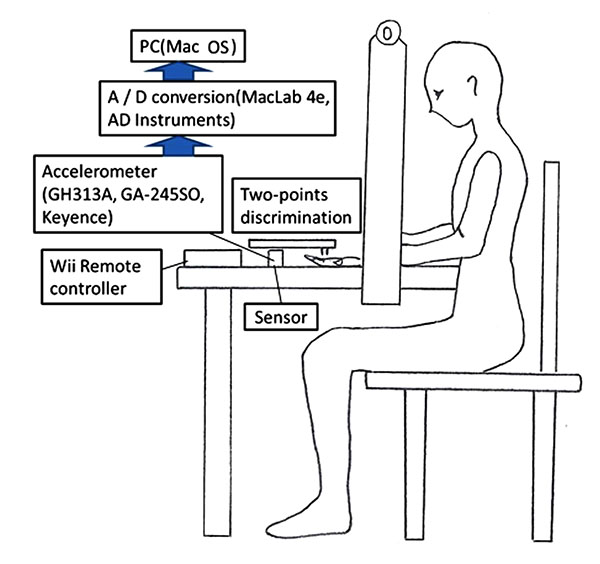
5. METHODS
5.1. Participants
Twelve male vocational college students participated in this experiment (mean age ± SD, 26.4 ± 12.11 years). All participants gave their informed consent and all experimental treatments conformed to the Declaration of Helsinki (1964).
5.2. Apparatus
Two-point discrimination threshold was measured using an electric digital caliper (cw-80216m, Freedom) attached to two metal shafts 0.2-mm in diameter. Vibrotactile noise was generated using the Wii Remote described in Experiment 1.
5.3. Procedures
Participants were seated at a table on which the vibrotactile stimulator was placed, with their left hand (non-dominant hand) placed palm upwards on the table. The experimental room was maintained at 25.0°C ± 0.85°C (mean ± SD). The parts of the fingers distal to the metacarpophalangeal (MCP) joints were flexed lightly (upward) so that the vibrotactile stimulus was applied only to the back of the hand. The distance between the Wii Remote and hand was about 10 cm, and the accelerometer was set at the mid-point. The visual field was partially restricted by a partition so that subjects could not see their hands (Fig. 3).
Two-point discrimination threshold was measured at the 3rd pad of the index finger (the palm-side skin surface at the proximal phalanx of the index finger) by the psychophysical constant method using six tip separation distances (1.5, 2.0, 2.5, 3.0, 3.5, and 4.0mm). In order to examine the effect of vibration noise on two-point discrimination, one of five noise intensities (S1–S5) or a no-noise condition (S0; control) was presented simultaneously with the tactile test stimuli.
The detection threshold of vibrotactile noise was pre-determined as S3 for each participant using the method of limits. Then, the other intensities were produced by decreasing (S4, S5) or increasing the number of cushioning sheet (S1, S2) in the same way in all participants.
Two-point discrimination threshold was measured during 2-s stimulus periods separated by 3-s response periods. A full session consisted of 60 measurement trials, and a total of 6 sessions were performed with an inter-session interval of 30s. The noise intensity was fixed during each session and was presented continuously throughout the session. The presentation orders of tip separation distance and noise intensity were quasi-random in each participant. A complete experiment lasted about 45 min.
5.4. Data Analysis
At each noise intensity level, tip separation distance for the 50% correct response rate (threshold) was calculated using the least-squares method. The obtained two-point threshold was normalized to the two-point threshold in the control condition (S0) to establish a uniform baseline across participants. From the normalized data, the median (±quartile) was calculated at each noise intensity level. A priori analysis using the sign test (one-sided) after Bonferroni correction was performed to test the effect of vibrotactile noise on two-point discrimination because the effect would be expected only for noise intensity around its detection threshold and because the two-point threshold could only decrease if the noise were effective.
6. RESULTS
The mean (±SE) noise threshold for all twelve participants was 1.4 ± 0.16V. The mean amplitude of vibration increased with intensity as adjusted by the number of cushioning sheets. The amplitude of vibration relative to threshold intensity at S3 was 0.6 at S1, 0.7 at S2, 1.5 at S4, and 1.6 at S5 (Fig. 4).
Two-point discrimination threshold in the control condition (S0) was 2.60 ± 0.26 mm (mean ± SE) at the 3rd pad of the index finger. Addition of a weak noise from the Wii Remote improved two-point discrimination, indicating successful induction of SR. Fig. (5) shows the change in two-point threshold with noise intensity. The two-point threshold decreased (improved) when a weak noise (S4) around its detection threshold (S3) was applied (0.90 ± 0.22 at S4 vs. 0.94 ± 0.22 at S3). Statistical analysis revealed a significant decrease in two-point threshold at S4 compared to control (p = 0.0215/2, r = 0.70), while more intense noise (S5) increased two-point threshold (i.e., reduced two-point discrimination). Less intense noise (S1 and S2) had no effect. Thus, the change in two-point discrimination exhibited a U-shaped relation with noise intensity, which is characteristic of SR.
7. DISCUSSION
7.1. Features of the Vibrotactile Stimulation System
The vibrotactile stimulation system developed here, has two remarkable features. One is stability of frequencies regardless of changes in amplitudes. As shown in Fig. (2), frequencies remained unchanged independent of changes in amplitudes. This is especially important in experiments, in which an independent variable is intensity of vibration. Another feature is that the present stimulator can provide vibration stimulus to a relatively wide area of the skin simultaneously, such as a whole area of the dorsal hand. Thus, we can examine the effect of remote vibration applied to a wide area of skin (not to a restricted area near the recording site) on the two-point threshold. The remote stimulation technique is especially important for the clinical use of SR to improve the two-point threshold, because sometimes actuators cannot be placed near the impaired site of patients.
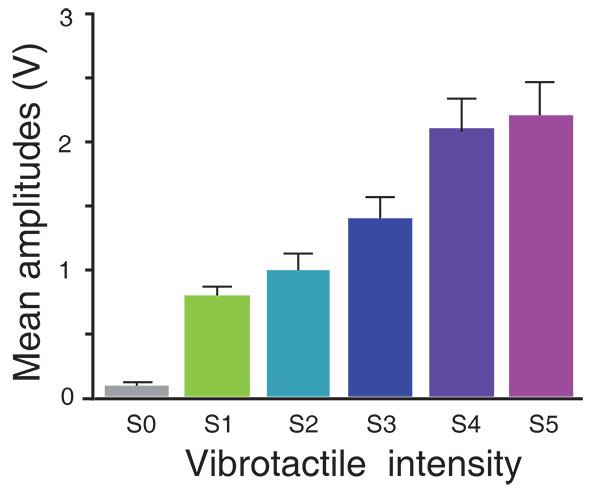
7.2. Mechanoreceptors Responsible for Two-point Discrimination
Cutaneous mechanical units in glabrous skin have been classified into four groups, fast adapting types I and II (FAI and FAII) and slowly adapting types I and II (SAI and SAII) that differ in receptive field size and stimulus response patterns [21, 22]. The receptive field sizes of FAI and SAI are small (12.6 and 11.0mm2, respectively), while the receptive field sizes of FAII and SAII are relatively large (101.0 and 59.0mm2, respectively). In addition, the receptive field border is clear for FAI and SAI but indistinct for FAII and SAII [23]. Spatial resolution is about 0.6mm−1 at the tip of the index finger, 0.25mm−1 at the third pad of the index finger, and 0.1mm−1 at the thenar eminence, acuities that parallel respective FAI and SAI receptor densities at each site, specifically 140 and 70 units/cm2 at the tip, 40 and 35 units/cm2 at the third pad, and 30 and 5 units/cm2 at the thenar eminence. In contrast, FAII and SAII receptor densities are constant across these sites. Therefore, FAI and SAI appear to be the mechanical units responsible for two-point discrimination by the index finger.
7.3. Frequency response Characteristics of the Cutaneous Mechanoreceptors
At least three cutaneous mechanical unit types respond to vibrational stimuli applied to the glabrous skin surface, SAI, FAI, and FAII. Of these, the threshold level is lowest for FAII [22]. In addition, the optimal frequency range is low for SAI and SAII, intermediate for FAI, and highest for FAII, with optimal frequency for FAII ranging from 100 to 300 Hz [22]. As the peak frequency of the vibrotactile stimulation system developed here was about 150Hz, it is likely that FAII was predominantly responsible for the vibrotactile response.
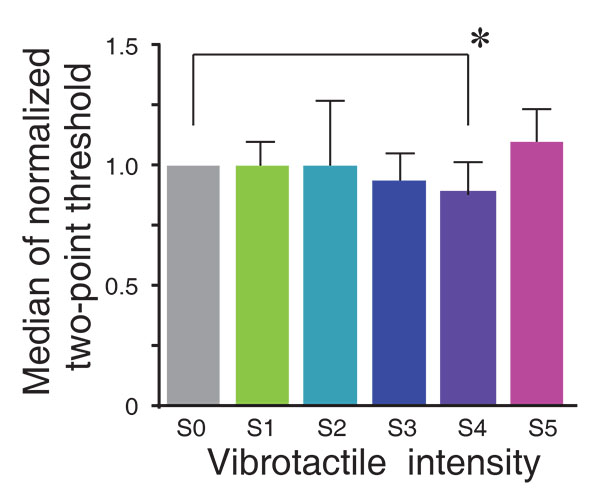
7.4. Subliminal Summation of Signal and Noise
Subliminal summation of signal and noise has been proposed to explain SR [24-27]. Noise is irregular in nature, so the sum of signal and noise is also irregular. Therefore, subliminal summation will exceed the detection threshold level in a stochastic manner when the noise intensity is weak. If noise intensity is too strong, however, the sum will always exceed the threshold. Conversely, if noise intensity is too weak, the sum will never exceed the threshold. Thus, an optimal (near-threshold) intensity must be present for SR to occur.
Little is known about the site of summation. Both external and internal SR has been demonstrated [28]. For internal SR, at least two sites are possible, receptors and the central nervous system (CNS) neurons [29]. In the present study, summation at the receptor level can be excluded because the mechanoreceptors responsible for signal and noise seems to be different. In addition, vibration amplitude decreases rapidly as it travels on the skin, with approximately 99% of the power lost over the first 6cm [30]. It thus appears more likely that the summation occurs at the cortical neuron level.
CONCLUSION
We have developed a new vibrotactile stimulation system consisting of a Wii Remote under PC control. In the first experiment, we examined the linearity and reproducibility of vibration amplitude and the stability of the frequency spectrum with stimulator (Wii Remote) activation intensity. Peak frequency was about 150Hz irrespective of intensity, suitable for FAII activation. This system is low-cost and useful for experiments using vibration stimuli, especially experiments using both subthreshold and suprathreshold stimuli.
In the second experiment, we evaluated the utility of this vibrotactile stimulation system by examining its effect on tactile sensitivity. Two-point discrimination threshold measured at the third pad of the index finger was improved when a weak vibrotactile stimulus (noise) was applied to the back of the same hand, while more intense and less intense vibrotactile noise did not improve tactile sensitivity. These results are consistent with the known behavior of SR in other sensory modalities and further suggest that remote application of vibrotactile noise can increase skin mechanical sensitivity at a distant site via SR. These results confirmed the accuracy and validity of our newly developed vibrotactile stimulation system.
ETHICS APPROVAL AND CONSENT TO PARTICIPATE
Not applicable.
HUMAN AND ANIMAL RIGHTS
No Animals/Humans were used for studies that are base of this research.
CONSENT FOR PUBLICATION
Not applicable.
CONFLICT OF INTEREST
The authors declare no conflict of interest, financial or otherwise.
ACKNOWLEDGEMENTS
Decleared none.